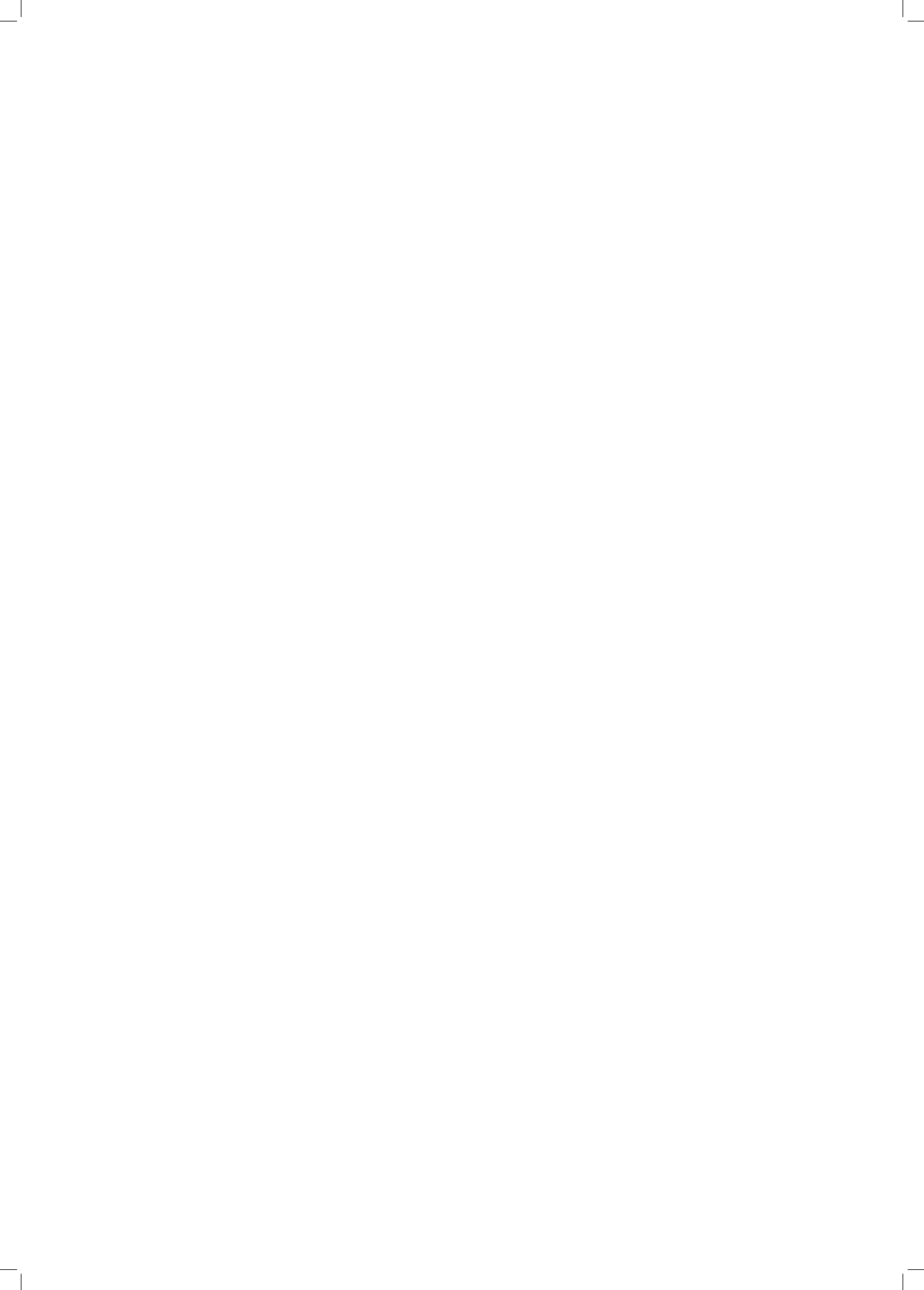
78 Leszek Orzechowski
Streszczenie
Celem publikacji było przedstawienie zagadnienia określania kosztów tworzenia ekstremalnej architektury kosmicznej za pomocą autonomicznych
robotów na przykładzie teoretycznego scenariusza załogowej misji na Marsa zawartego w dokumencie NASA Mars Reference Mission. Autor opi
suje strategie pozwalające określić energię ucieleśnioną zawartą w architekturze stworzonej z lokalnych materiałów za pomocą addytywnych pro
cesów produkcyjnych, czyli druku 3D. Energia ucieleśniona jest sumą energii włożonej w proces wytworzenia dowolnej usługi bądź dobra, w tym
energii zużytej do pozyskania surowców do jego wytworzenia, transportu. W ramach omawianej przez autora strategii zakresy prac oraz zadań
zostały pogrupowane w cztery działy pokrywające się ze strukturą rozgrywki strategicznych gier komputerowych z gatunku 4X. Gry komputerowe
4X biorą swoją nazwę od skrótowego opisu elementów prowadzenia rozgrywki: explore, exploit, expand, exterminate, co w wolnym tłumaczeniu
znaczy: eksploracja, eksploatacja, ekspansja, eksterminacja. W trakcie trwania gry użytkownik skupia się na zarządzaniu i rozwijaniu cywilizacji
lub miasta, gdzie głównym jego zadaniem jest pozyskiwanie i zarządzanie zasobami. Taka struktura została uznana przez autora za obiecujący punkt
wyjścia strategii optymalizacji procesu budowy architektury marsjańskiej przez agentów robotycznych. Wspomniana symulacja mogłaby zostać
użyta do stworzenia narzędzia do optymalizacji procedur na potrzeby realnej misji na Marsa przy wykorzystaniu jako wyznacznika energii uciele
śnionej. Takie narzędzie miałoby szanse obniżyć koszty podczas pierwszych załogowych misji na inne planety. W dalszej perspektywie stosowanie
takiego rozwiązania do gromadzenia danych o energii ucieleśnionej pozwoli na zachowanie całości danych o odcisku ekologicznym kolonizacji Marsa.
Słowa kluczowe: architektura kosmiczna, druk 3D, energia ucieleśniona, automatyzacja budowy
Abstract
The purpose of this publication is to present the problem of determining the costs of creating extreme space architecture using autonomous robots
based on the example of the theoretical manned mission to Mars scenario contained in the NASA Mars Reference Mission document. The author
focuses on strategies that can determine the embodied energy contained in architecture made from local materials, using additive manufacturing
processes – 3D printing. Embodied energy is the sum of the energy invested in the process of producing any service or good, including the energy
consumed to obtain raw materials for its production and transport. Procedures to optimise the work of robotic agents will be crucial during manned
Mars missions with limited access to resources, including electricity. Using robots to work on the surface will precisely determine the amount of
energy used to complete the given job, which will enable optimisation and the determination of the embodied energy of these works. As part of the
strategy discussed by the author, the scope of work and tasks are grouped into four divisions that coincide with the gameplay structure of 4Xtype
strategic computer games. 4X computer games get their name from the brief description of the gameplay elements: “explore, exploit, expand, exter
minate”. During the game, the user focuses on managing and developing a civilisation or city, where resource acquisition and management play
a central role. The author considers such a structure to be a promising starting point for the strategy of optimising the process of building a Martian
architecture using robotic agents. Procedures that can determine the embodied energy of the described processes have been grouped into the sections
Exploration, Extraction, Exploitation, and Expansion, and have been included in the simulation model for the manned mission to Mars in the form
of a 4Xtype computer game prototype. This simulation could be used to create a tool for optimising procedures for the needs of an actual mission
to Mars, using embodied energy as an indicator. Such a tool would have the potential to reduce costs during the first manned missions to other
planets. In the longer term, the use of such a solution for the collection of data on embodied energy will preserve all environmental footprint data
of the colonisation of Mars.
Key words: space architecture, 3D printing, embodied energy, building automation
[11] ESA, http://www.esa.int/Highlights/Lunar_3D_printing, 2015 [acces
sed: September 2017].
[12] NASA 3D-Printed Habitat Challenge, https://americamakes.us/
wpcontent/themes/3dchallenge2015/assets/Design_Competition_
Rules.pdf, 2015 [accessed: September 2017].
[13] Huntsberger T., Pirjanian P., Schenker P.S., Robotic outposts as pre-
cursors to a manned Mars habitat, „AIP Conference Pro ceed ings”
2001, Vol. 552, Iss. 1, https://www.researchgate.net/publication
/2801344_Robotic_Outposts_as_Precursors_to_a_Manned_Mars_
Habitat [accessed: September 2017].
[14]
Huntsberger T., Rodriguez G., Schenker P.S., Robotics challenges for
robotic and Human Mars Exploration, Jet Propulsion Labo ra tory,
Pasadena 2012, http://citeseerx.ist.psu.edu/viewdoc/download?doi=
10.1.1.83.3242&rep=rep1&type=pdf [accessed: September 2017].
[15]
Fong T., Thorpe C., Baur C., Collaboration, Dialogue, and Hu man-
Robot Interaction, [w:] 10
th
International Symposium of Robotics Re -
search, Lorne, Victoria, Australia, SpringerVerlag, Pitts burgh, PA
2001, https://pdfs.semanticscholar.org/f617/005acc94c64f6f8fed991f
9949774257ae8f.pdf [accessed: September 2017].
[16] Space is More design team, www.spaceismore.com [accessed: Sep
tember 2017].
[17] 4x Strategies, https://en.wikipedia.org/wiki/4X [accessed: Sep tem
ber 2017].
[18]
Civilization, https://en.wikipedia.org/wiki/Civilization_(video_game)
[accessed: September 2017].
[19] Colonization, https://en.wikipedia.org/wiki/Sid_Meier%27s_
Colonization [accessed: September 2017].
[20] Pharaoh, https://en.wikipedia.org/wiki/Pharaoh_(video_game)
[accessed: September 2017].
[21]
Master of Orion II, https://en.wikipedia.org/wiki/Master_of_Orion_II
[accessed: September 2017].
[22] Meyer Th.R., McKay C., The resources of Mars for human settle-
ment, „Journal of the British Interplanetary Society” 1989, Vol. 42,
147–160, https://www.researchgate.net/publication/252478654_
Using_the_Resources_of_Mars_for_Human_Settlement [acces
sed: September 2017].
[23] Schwenzer S.P., Abramov O., Allen C.C. et al., Gale Crater: For-
ma tion and postimpact hydrous environments, „Planetary and Space
Science” 2012, No. 70, Iss. 1, 84–95, http://www.sciencedi rect.
com/science/journal/00320633/70 [accessed: September 2017].
[24] Golombek M., Grant J., Vasavada A.R. et al., Final Four Landing
Sites For The Mars Science Laboratory, LPI Contribution No. 1608,
1520, Texas 2011, https://www.lpi.usra.edu/meetings/lpsc2011/pdf/
1520.pdf [accessed: September 2017].
[25] Arvidson R.E., Bellutta P., Calef F. et al., Terrain physical proper-
ties derived from orbital data and the first 360 sols of Mars Science
Laboratory Curiosity rover observations in Gale Crater, „Journal
of Geophysical Research: Planets” 2014, Vol. 119, 1322–1344,
http://onlinelibrary.wiley.com/doi/10.1002/2013JE004605/epdf
[accessed: September 2017].
[26] Milliken R.E., Ewing R., Fischer W., Hurowitz J., Clay and Sul-
fate-Cemented Sandstones in Gale Crater: Evidence from Orbital
Data, [w:] 44
th
Lunar and Planetary Science Conference, The
Wood lands, Texas, March 18–22, 2013, 1243, https://www.lpi.usra.
edu/meetings/lpsc2013/pdf/1243.pdf [accessed: September 2017].
[27] Milliken R., MSL Science Team, Mineral Mapping of Gale Crater
Using Orbital Data: Results From Visible Near Infrared Reflec-
tance Spectroscopy, „Search and Discovery” 2014, Article #51013,
http://www.searchanddiscovery.com/documents/2014/51013milliken
/ndx_milliken.pdf [accessed: September 2017].