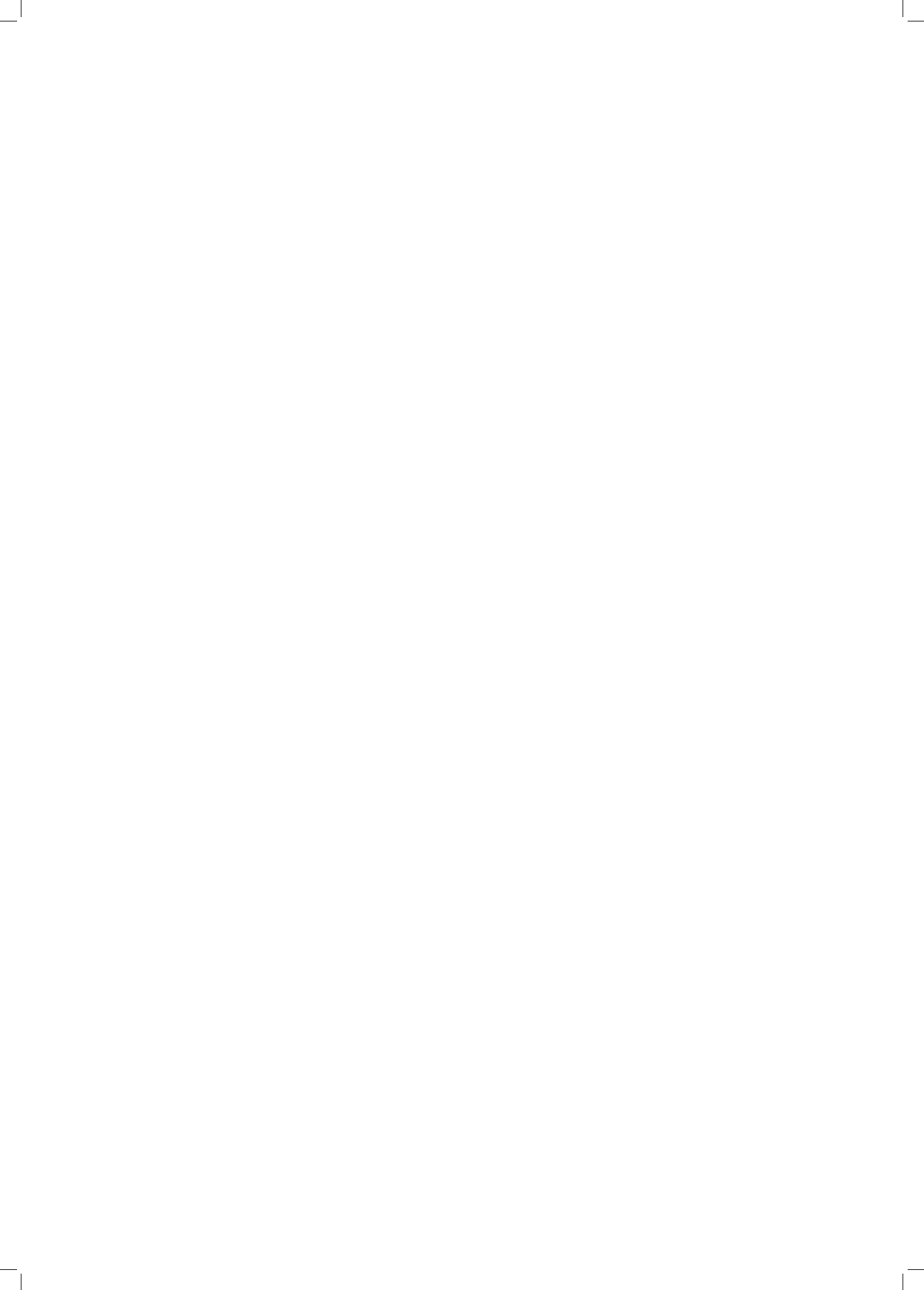
Termomodernizacja budynków drewnianych/Thermal performance improvement of wooden buildings 105
Podstawowym budulcem stosowanym do wznoszenia
oma wianych konstrukcji było lite drewno pozyskiwane
z drzew iglastych, głównie świerka, sosny i jodły, a także
modrzewia, sporadycznie z liściastego drewna bukowego.
Drewno stanowiące element konstrukcji stale pracu-
je, na skutek wysychania kurczy się, wraz ze wzrostem
wilgotności pęcznieje. W wyniku zmniejszającej się ob-
jętości włókien drewna ściana wieńcowa osiada. W roz-
wiązaniach tradycyjnych ścian wieńcowych pomiędzy
poszczególnymi belkami powstają poziome szczeliny.
Taki stan powoduje niekontrolowany przepływ powietrza
przez ściany zbudowane z jednej warstwy materiału. Ten
niekontrolowany przepływ powietrza intensykują także
głębokie podłużne spękania drewna skierowane ku jego
rdzeniowi. Pęknięcia stanowiące poważną wadę materiału
pogarszają jego właściwości mechaniczne i ciepłochron-
ne, przyspieszają wnikanie wody i zarodników grzybów
do wnętrza drewna.
System elementów budujących przegrody zewnętrzne
(ściany wieńcowe i ryglowe) powinien być mało prze-
puszczalny dla powietrza. Dotyczy to również spoin
i styków. Zbyt intensywna wymiana powietrza przez nie-
szczelności w jednowarstwowych przegrodach wywołuje
nadmierne konwekcyjne straty ciepła. Wzrost zawilgoce-
nia drewna spowalnia proces przepuszczania gazów przez
materiał, intensykuje jednak wymianę ciepła.
Przewodność cieplna i izolacyjność
ścian drewnianych
Wymiana ciepła i pary wodnej przez przegrody ze-
wnętrzne jest procesem złożonym. Przewodność cieplna
materiału opisuje jego zdolność do przewodzenia ciepła.
Jej miarą jest współczynnik przewodzenia ciepła (λ).
Drew no, w porównaniu z takimi materiałami jak beton,
ka mień czy stal, ma względnie małą przewodność. Jej
wielkość zależy od gęstości objętościowej (gatunku drew-
na), wilgotności i temperatury materiału. Przepływ ciepła
wyraźnie intensykuje się wraz ze wzrostem wilgotności,
temperatury i gęstości drewna [6].
Wieńcowe ściany zewnętrzne wykonane z litego drew-
na przy szerokości bali około 25 cm nie spełniają wyma-
gań dotyczących odpowiedniej wartości współczynnika
przenikania ciepła (obliczanego wg PN-EN ISO 6946).
Od 2014 r. systematycznie wzrastają wymagania od-
nośnie do oszczędzania energii i izolacyjności cieplnej
przegród. Obecnie maksymalna wartość wskaźnika EP,
określającego roczne zapotrzebowanie obiektu na nieod-
nawialną energię pierwotną, oraz wartość współczynnika
prze nikania ciepła – U (dla ścian zewnętrznych) dla bu-
dynku jednorodzinnego wynosi odpowiednio 95 kWh m
2
× rok oraz 0,23 W/m
2
× K, a od 2021 r. będzie wynosić
70 kWh/m
2
× rok oraz 0,20 W/m
2
× K.
Przeprowadzone badania terenowe wykazały, że ogólny
stan techniczny budynków o konstrukcji drewnianej, przy-
słupowej, liczących kilkadziesiąt, a nawet więcej lat jest
w większości niezadowalający. Mimo że ściany wykona-
ne z litego drewna mają dużą pojemność cieplną (dzię-
ki
czemu domy te zużywają mniejszą ilość energii, niż wy-
nikałoby to tylko z szerokości przegród drewnianych [7]),
horizontal gaps between the logs appear in the traditional
corner-notched log walls. This, in turn, results in uncon-
trolled air penetration through single layer walls. This
uncontrolled air penetration is further increased by deep,
longitudinal cracks in the wood going toward its core. The
cracks which constitute serious material defects decrease
its mechanical and thermal properties and they increase
the penetration of water and fungal spores inside the wood.
The system of elements composing the outer space di-
viders (corner-notched log walls and half-timbered walls)
should provide for poor air penetration. This also applies
to joints and contact places. Too intensive air exchange
through the gaps in single-layer space dividers causes ex-
cessive convective losses of heat. An increase in moisture
in wood slows the process of gas penetration through the
material and at the same time intensies the heat exchange.
Thermal conductivity and thermal performance
of wooden walls
The exchange of heat and water vapor through outer
space dividers is a complex process. The thermal conduc-
tivity of material denes its ability to transfer heat. That
conductivity is measured by the heat transfer coefcient
(λ). Compared with the material, such as concrete, stone
or steel, wood demonstrates a relatively low conductivity.
Its rate depends on the density (wood type), moisture and
temperature of the material. The heat transfer evidently
grows along with the increase of wood moisture, tempera-
ture, and its density [6].
External corner-notched log walls made of solid tim-
ber where the logs are about 25 cm wide do not meet the
requirements regarding adequate level of the heat transfer
coefcient (per PN-EN ISO 6946).
The requirements regarding energy efciency and
thermal performance of space dividers have been stead-
ily growing since 2014. Currently, the maximum value
of EP coefcient, which denes the annual demand for
non-renewable sources of primary energy and the value of
heat permeability coefcient – U (for external walls) for
a single-family building, is respectively 95 kWh/m
2
× year
and 0.23 W/m
2
× K, and from 2021 it shall be 70 kWh/m
2
× year and 0.20 W/m
2
× K.
Field tests have demonstrated that the general tech-
nical condition of most wooden buildings with a corner
timbered-framework which are several dozen years old or
even older is unsatisfactory. Although the heat capacity of
their walls, which are made of solid wood, is high (which
is why the houses use less energy than it would seem,
judging only by the thickness of the wooden space divid-
ers [7]), in the face of constantly growing requirements,
in the opinion of the authors, the thermal performance im-
provement of outer space dividers is fully justied.
The directions
of thermal performance improvement
If they are supposed to be used further on, the wooden
enclosed structures with a corner timbered-framework
should undergo a thorough renovation. In regards to the